RED.3 Battery system with cells of type 21700
The new battery system is equipped with automotive cells of the 21700 standard. A battery block is made up of 14 cells in the standard version and 18 cells in the version with increased capacity. In Antares aircraft, 36 of these battery blocks are arranged together in one wing, forming a battery chain.

Services, variants and conversion
The ANTARES.RED.3 battery system is offered in S-tandard and L-arge variants. The S variant can be retrofitted to older Antares aircraft and delivers a climb altitude of 4,200 m ( 13800 ft )and a range of 280 km (150 nautical miles) in sawtooth flight. Battery chains can be easily retrofitted through large openings in the wing root rib.
Variant L is to be implemented preferably in new aircraft, since four more cells are installed per battery block, thus increasing the overall dimensions of the battery chain. The additional weight is 11kg per battery chain. The total 22 kg increased mass is not critical for the Antares and a small price to pay for the then possible climb altitude of 5600 m ( 18370 ft ) or 380 km ( 205 nautical miles ) range in sawtooth style after self-launch.
Certification, safety and reliability
Ever since we began developing electric drives 25 years ago, our focus has been on safety and reliability. The drive system was developed and approved in accordance with recognized standards (ED-79, DO-178C and DO-254 with Design Assurance Levels (DAL) C) for the development of safety-critical hardware and software.
The reliability of our developments is also demonstrated by the 160,000 trouble-free flight hours of the Antares fleet.
Battery chain in the wing
The battery chain is carried in a rail system that is glued into the wing structure. The individual modules are hinged together so that any deflection of the wing is not imposed on the modules. The entire battery chain is only rigidly connected at the wing root rib. The free outer end can move in the guide rails when the wing deflects.
Thermal and electrical monitoring of the battery cells
When charging and discharging the drive battery, it is important to comply with certain temperature ranges. This ensures that the specified amount of energy can be absorbed and that maximum energy extraction is possible.
The temperature is measured at each individual cell and displayed and monitored on the specially developed ECDS 4 control system. If a temperature falls below the recommended charging or discharging temperature, the integrated battery heater is automatically switched on. If exceeded, heat can be dissipated via ventilation flaps.
Single-cell monitoring is part of the “Protect Ahead” safety concept, which enables incorrect thermal behavior to be detected at an early stage. In the event of a “thermal runaway”, the hot gases generated can escape from the ventilation flaps and do not damage the aircraft. The replacement of individual defective battery blocks is possible.
Unavoidable tolerances in the manufacturing process of the cells can lead to an uneven self discharge. This results in variances in the individual cell voltages. In order to consistently utilize the full capacity of the drive battery, these voltage differences must be counteracted. Therefore, the voltage of each individual cell is also measured and monitored. The control system is informed about the cell voltages and can automatically perform a balancing process. Charging, discharging and balancing procedures can be controlled via a digital communication module.
Voltage levels
The single cell should not be discharged below a voltage value of 3V to avoid increased aging and damage to the cell. The upper limit for a charged cell is 4.2 volts. This results in a voltage range for normal operation of 216 volts to 302 volts. In an emergency, the pilot can of course decide to discharge the battery system beyond the limits specified here.
Information about the status of the battery system is shown on a large full-color display. Cautions and warnings are also communicated with an audio announcement. The decisive factor here is the individual cell voltage. Differences in individual cell voltages result from manufacturing tolerances and can significantly reduce the usable capacity of the battery system. Therefore, the Antares battery system has an electronic system that automatically adjusts the cell voltages of the individual cells. This process is called “balancing”. The balancing system limits the voltage difference to a maximum of 20 millivolts, allowing the full battery capacity to be used at all times.
Battery heating
The performance of the whole battery depends on the battery temperature. The normal temperature range at which the battery can provide its full energy is 20 -30 °C ( 68 – 86 °F ). If the temperature falls, the ability to release energy also falls. When charging the battery, the amount of energy stored also depends on the battery temperature. If the battery is charged at a temperature below 20°C, the full capacity of the battery will not be available.
For this reason, each battery module is equipped with a heater. Before takeoff and before each charging process, the battery can automatically be heated to the optimum temperature range. During flight, the battery management system ensures that the battery remains within the optimum temperature range.
Life expectancy
The factors influencing the service life of a battery are the number of cycles and calendar aging. A very long battery life expectancy can be achieved by selecting the right cell chemistry and battery design, as well as by operating and storing the battery within specific temperature and voltage ranges.
Based on our many years of experience with the previously used cell from the manufacturer SAFT, the service life of the Antares battery system is not limited by the number of cycles, but by the calendar ageing of the cell. After 20 years, this battery still has a capacity of 80% of its original value, but it should be replaced for reasons of safety.easons.
The new battery system uses cells of the 21700 format. Even with the selected battery cell, even for intensive use, it is not the number of cycles that is relevant, but the calendar aging.
Future development
One advantage of this cell format is that it is a common industrial format for high-quality applications such as in the automotive industry. Continuous further development of the 21700 battery cell format can therefore be expected, with a generation change approximately every three years.
By upgrading the Antares.RED battery system with future cell generations, the power reserves of the Antares will continue to grow. One benchmark for innovation in Antares aircraft is the increase in gravimetric energy density (see graph). This describes how much energy per weight can be stored in a battery. The higher the value, the more energy can be stored and installed in Antares aircraft while maintaining the same battery mass and battery cell dimensions. It can be deduced from this that an Antares with modern RED battery systems will be able to climb ever higher in the future. Today, the L variant of Antares.RED.3 already reaches 5,600 m (18370 ft ). If the development trend is followed, altitudes of up to 6,700 m ( 22000 ft ) will be feasible by 2030.
Thanks to the large quantities required, the battery cells can be procured in very high quality at reasonable prices. As the wear-free electric motor does not cause any reduction in value, optimum resale values can also be achieved in the future. This provides a high level of investment security. In the near future, combustion engines will no longer play a role in soaring.
Gravimetric energy density
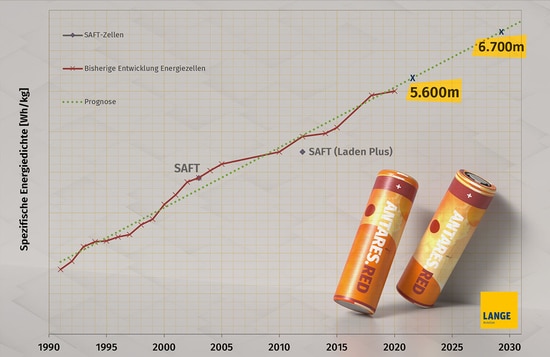